What do adhesives, light displays, gasoline, and industrial rubber have in common? They all have the simplest organic alcohol — methanol — as their foundation. Methanol is a building block for a wide range of chemicals, and with one of the highest hydrogen-to-carbon ratios, it shows potential as a high-energy-efficiency fuel. Methanol also accounts for 28% of the emissions associated with primary chemical production. Methanol is mainly produced from natural gas (gray methanol) in a two-step process — natural gas reforming into synthetic gas (syngas) and catalytic conversion of syngas into methanol — and has a production cost of roughly USD 160/tonne. But growing applications for methanol as a low-carbon fuel and a carbon carrier for the chemicals industry require a transition away from fossil feedstock.
This transition, however, comes at a cost. And the cost grows steeper the further we move from incumbent feedstock and incumbent processes. We look at the cost of producing three different grades of low-carbon methanol (blue methanol, biomethanol, and e-methanol) in terms of how much they deviate from the incumbent gray methanol pathway.
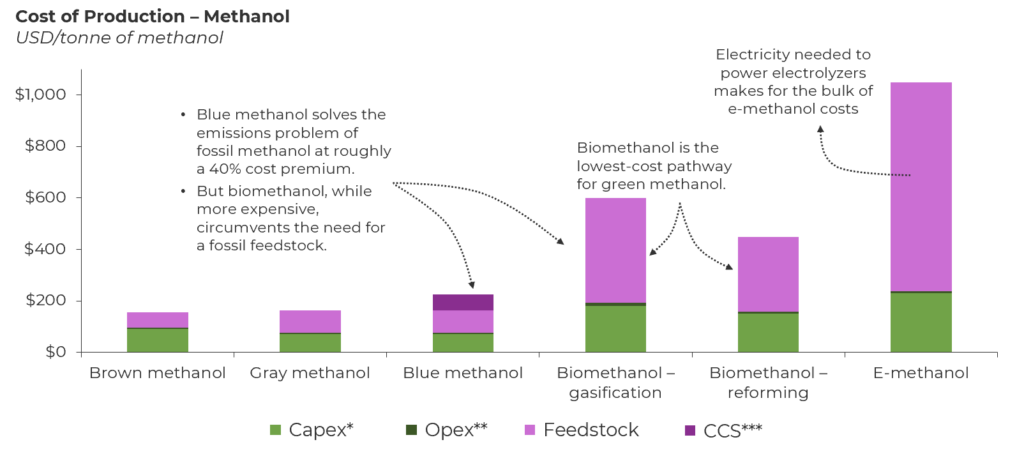
*Capex, capital expenditures, **Opex, operating expenditures, ***CCS, carbon capture and storage
- Blue methanol (identical feedstock, identical process). The first adjacency to gray methanol is blue methanol, which retains the incumbent natural gas pathway with a carbon capture retrofit. Blue methanol costs USD 230/tonne of methanol and allows operating facilities to reduce the carbon intensity of gray methanol by approximately 40% via carbon capture. Activity here has been sparse, but earlier this year, the U.S. saw an announcement about a USD 3 billion project at the Port of Lake Charles to produce blue methanol and other chemicals. However, this is not a long-term solution because blue methanol will eventually be penalized in its lifecycle analysis since the carbon embedded is still from natural gas and, therefore, fossil derived. Emissions (including methane) from feedstock sourcing and gas flaring result in an unavoidable carbon intensity that can hinder market adoption.
- Biomethanol (new feedstock, similar process). The next adjacency involves switching over to a nonfossil carbon source, but still retaining some aspects of the incumbent natural gas process. The nonfossil carbon source is biomass or biogas, and the process aspect being retained is the syngas intermediate. Solid biomass feedstock like wood pellets or municipal solid waste can undergo gasification, and a gaseous feedstock like biogas can undergo reforming to produce syngas. Syngas is then converted into biomethanol, which is chemically identical to fossil methanol. The challenge in this process is not syngas conversion but rather syngas production from biomass. Biomass is more expensive than natural gas, and the need for large gasifiers to ingest high volumes of biomass drives up the capex. This results in an overall cost of approximately USD 600/tonne of biomethanol. As we look ahead, scale can help drive down costs, but biomass prices will be heavily impacted by price inflation stemming from regional supply limitations. Reforming biogas is slightly less expensive, approximately USD 450/tonne of biomethanol, but the challenge here lies in the biogas being used directly for power generation instead of upgraded into grid-quality renewable natural gas that can be used for biomethanol production. This is more prominent in regions like Europe, which strives for energy security in times of geopolitical conflicts. Overall, biomethanol is the cheapest grade of nonfossil methanol but will face a feedstock issue in the long term to completely supplant fossil methanol.
- E-methanol (new feedstock, new process). The last option is tapping into captured CO2 and green hydrogen, which involves a new technology — CO2 hydrogenation — that differs from converting syngas into methanol. E-methanol has the most favorable carbon footprint, and one can argue (and hope) that the abundance of carbon capture and green hydrogen in 2050 will render these new feedstocks virtually limitless and inexpensive. But we are far from that reality. Producing 1 tonne of e-methanol requires 0.2 tonne of hydrogen, and an optimistic USD 4/kg cost of green hydrogen translates to a hydrogen feedstock cost of USD 800/tonne of methanol. Therefore, the overall e-methanol cost today stands close to USD 1,000/tonne of methanol, with its long-term trajectory aligned with developments in the carbon and hydrogen economies. However, many chemical majors are providing state-of-the-art catalysts and working with value chain partners to set up first-of-a-kind e-methanol facilities that can be replicated.
Scaling e-methanol to drive down costs: Fixed and varied value chain partners
Scaling up e-methanol facilities will require setting up standardized and replicable commercial facilities of similar production capacities. Doing this will require strong technology partners (beyond catalyst developers) that can provide standardized and replicable technology components. We specifically call out Liquid Wind’s approach in the context of future cost reductions through scale. Aiming to set up 80 e-methanol facilities in the Nordics, each with a capacity of 100 ktonne/y, Liquid Wind has fixed its technology providers — Carbon Clean for carbon capture, Siemens for green hydrogen, and Topsoe for the catalyst — and varied its utility partner based on the location. Over time, this approach creates efficiencies and learnings in integrating distinct technologies as opposed to using new capital to optimize and refine technology components.
Beyond CO2 hydrogenation: What else can be done?
E-methanol is still an emerging technology, but at Lux, we always have our eyes on the horizon for what’s next. While we do expect e-methanol projects involving chemical majors to have the highest chance of commercial success, the startup ecosystem for e-methanol is still ripe with innovation. Here are a few other approaches to producing e-methanol:
- Methanology. An enzyme-based electrochemical process that converts CO2 and water into methanol; no hydrogen required.
- Oxylus Energy. Direct CO2 electrolysis into methanol using a novel cobalt-on-carbon catalyst.
- ICODOS. Continuous carbon capture and conversion process that is retrofitted into an industrial facility and produces e-methanol onsite. The cost savings here come from reduced carbon capture costs (owing to energy savings from desorption and CO2 compression) and capex but still requires green hydrogen.